
Second, a major difference between the changes in the K + channels and the changes in the Na + channels is that the K + channels are slower to activate or open. These K + channels are normally closed, but open in response to depolarization. There are two important points.įirst, just as there are channels in the membrane that are permeable to Na + that are normally closed but then open in response to a voltage, there are also channels in the membrane that are selectively permeable to K +. The figure shown to above indicates the changes in K + conductance as well as the Na + conductance. These changes can be measured with the voltage-clamp technique as well. In addition to voltage-dependent changes in Na + permeability, there are voltage-dependent changes in K + permeability. However, inactivation is not enough by itself to account fully for the repolarization. Inactivation contributes to the repolarization of the action potential. The Na + channels begin to close, even in the continued presence of the depolarization. Note that the permeability increases rapidly and then, despite the fact that the membrane potential is clamped, the permeability decays back to its initial level. Thus, the experiment provided support for the existence of voltage-dependent Na + channels.įigure 2.4 also indicates an important property of the voltage-dependent Na + channels. The more the cell is depolarized, the greater is the Na + conductance. Hodgkin and Huxley clamped the membrane potential to various levels and measured the changes in Na + conductances (an electrical term for permeability, which for the present discussion can be used interchangeably). The amount of current necessary to stabilize the potential can then be used to quantify membrane permeability.
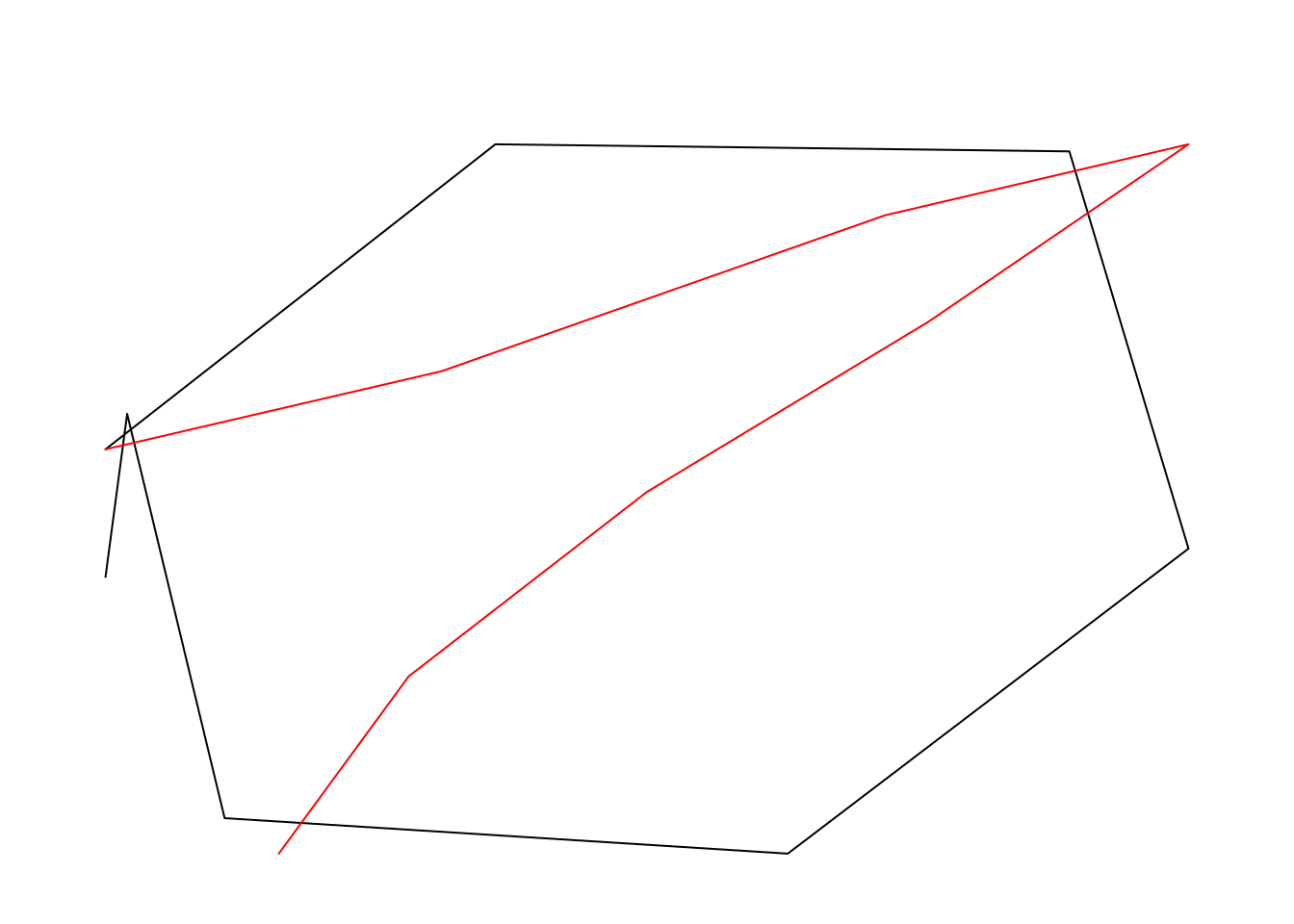
An electronic device known as a voltage-clamp amplifier can "clamp" or stabilize the membrane potential to any desired level and measure the resultant current required for that stabilization. In order to test the Na + hypothesis for the initiation of the action potential, it is necessary to stabilize the membrane potential at a number of different levels and measure the permeability at those potentials. Once some critical level is reached a positive feedback or regenerative cycle will be initiated, causing the membrane potential to depolarize rapidly from -60 mV to a value approaching the Na + equilibrium potential. The key point is that the increase in Na + permeability would produce a greater depolarization, which will lead to an even greater number of Na + channels opening and the membrane potential becoming even more depolarized.

Suppose a small depolarization causes some of the Na + channels to open. This simple hypothesis of voltage-dependent Na + channels goes a long way toward explaining the initiation of the action potential. They suggested that the properties of some Na + channels in nerve cells (and muscle cells) were unique in that these channels were normally closed but could be opened by a depolarization. How is it possible for a cell to initially have a resting potential of -60 mV and then, in response to some stimulus (a brief transient depolarization which reaches threshold), change in less than one millisecond to having a potential of approximately +40 mV? In the 1950's, Hodgkin and Huxley, two British neurobiologists, provided a hypothesis for this transition. The peak of the action potentials approaches but does not quite reach ENa, because the membrane retains its permeability to K +. If alpha is very large, the Na + terms dominate, and according to the GHK equation, the membrane potential will move towards the Na + equilibrium potential. In principle, any point along the trajectory of action potential can be obtained simply by varying alpha in the GHK equation. Note that the resting potential is not equal to the K + equilibrium potential because, as discussed previously, there is a small resting Na + permeability that makes the cell slightly more positive than EK. An action potential is bounded by a region bordered on one extreme by the K + equilibrium potential (-75 mV) and on the other extreme by the Na + equilibrium potential (+55 mV).
